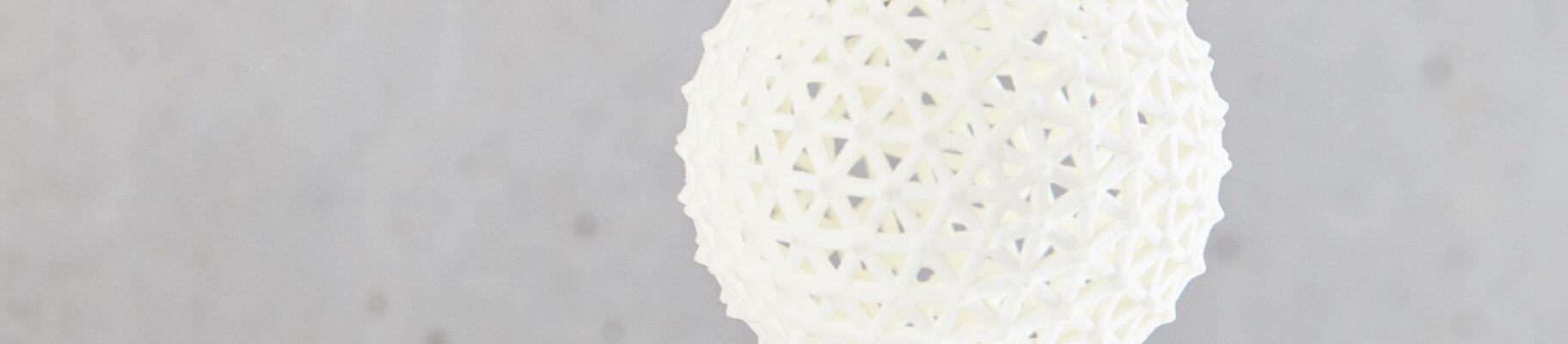
New to AM: A Beginner's Guide to Additive Manufacturing and its Major Technologies
MAY 8, 2023 | Reading time: 5 min
Are you new to the exciting world of additive manufacturing and the key technologies that make it happen? We've put together a comprehensive but straightforward guide in this blog.
Additive manufacturing is future-focused manufacturing
Although it’s not a new technology in the strictest sense, additive manufacturing (AM) — sometimes referred to as 3D printing — has attracted significant attention in recent years. And there are many exciting reasons for that:
-
Whether an organization is using AM systems and materials to shape cutting-edge polymer blends or versatile aluminum alloys, the fact remains that it has equipped itself to create parts according to geometric parameters that were once impossible or (at the very least) extremely difficult to achieve.
-
Parts made through additive manufacturing may appear "simpler" than their traditionally manufactured counterparts — smaller, more lightweight, requiring fewer individual components and so on. But in numerous cases, an AM-fabricated part can either match or beat the traditional part's performance.
-
Additive manufacturing is proving its mettle in numerous applications. These range from the casual and fun (running shoes and hockey helmets) and the wildly exciting (rocket engines) to the truly important (medical implants and surgical equipment).
-
Adopters of additive manufacturing have seen tangible increases over time in production quality and efficiency, alongside reductions in time-to-market and overall expenses.
EOS has been part of the additive manufacturing revolution since the technology's earliest days, developing everything from 3D printing systems and software to educational and consulting services. But if you're truly new to AM, you may not want to be sold on our organization's bona fides just yet — you want to learn more about the background, processes, applications and successes of this remarkable technology.
And that is exactly the journey we intend to take you on.
A brief history of additive manufacturing
In some sources, you'll see a patent filed by American engineer and inventor Bill Masters — specifically U.S. 4665492A, dated July 2, 1984 — called the first 3D printing patent. Others award this distinction to a Charles Hull patent filed in the U.S. that same year. And both are crucial to the technology's history.
But a patent application filed with the Japan Patent Office (JPO) by Hideo Kodama (JP S56-144478) predates Masters and Hull by almost three years: Like the application Masters eventually sent to the U.S. Patent and Trademark Office (USPTO), it describes an automated process for creating 3D shapes by using heat — either laser or ultraviolet (UV) — to harden soft plastics.
Kodama couldn't attract funding for his idea, despite publishing it in well-known trade journals, so he abandoned his patent application. Masters went through his patent, received a grant and worked in the field for about 10 years but also attracted little attention.
An unsung watershed year
1984 marked the invention of several pivotal AM processes — though it took time for these early innovations to be realized at scale. Masters' design (like Kodama's) involved early examples of computer numerical control (CNC) and computer-aided design (CAD) programming integral to future AM systems.
This year also saw two patent filings vital to one of the first additive manufacturing processes with potential commercial viability: stereolithography (SLA).
The first, by French inventors Alain le Méhauté, Olivier de Witte and Jean Claude André, was abandoned by their sponsor soon after its filing. The second, belonging to Charles Hull, prompted production of the SLA-1 3D printer. The SLA-1 was prohibitively expensive for many, and Hull, like other early AM advocates, had trouble convincing traditional manufacturers of its potential. However, Hull also invented STL for the SLA-1's design files, and that format remains common today.
Refinement and upscaling
Engineers, including those at EOS, spent the 1990s steadily improving early additive manufacturing processes — SLA and fused deposition modeling (FDM) — and developing new ones. Most (though not all) focused on shaping polymers into 3D components, including material and binder jetting, sheet lamination, laser powder bed fusion (LPBF) and selective laser sintering (SLS). Some of these, like SLS and LPBF, allowed advanced metalworking but meant to do so for traditional (subtractive) manufacturing. But the development of material deposition methods including microcasting and thermal spray paved the way for the additive use of metal.
Coupled with advancements in CAD software and the rise of powdered polymers and metals, the refinement of industrial 3D printing systems helped them become well-known for rapid prototyping throughout the 2000s. In the 2010s, additive manufacturing scaled up enough to facilitate part assembly at much higher levels, in some cases enough so to be accurately considered "mass production." Even with the few limitations still affecting AM — material compatibility (mostly on the metal side), the initial cost and efforts needed for implementation, a skills gap (albeit a shrinking one) and skepticism from traditional manufacturers — the technology's presence and importance continue to expand.
The Major AM Technologies
The next step in any journey toward serious plans to implement additive manufacturing in your production facilities is learning about the fundamentals of key AM technologies.
This isn't a list of every single additive manufacturing method, and not all of the techniques detailed below are used in EOS systems. But making the most informed decision possible about AM requires an understanding of the topic that extends beyond our product catalog.
Vat photopolymerization
While not the SLA process still in use today, vat photopolymerization (or sometimes simply polymerization) is arguably the most common. This method's name comes from the source of the material that fuels production: a vat of liquid photopolymer resin. A "build platform" is repeatedly lowered into (and then raised from) the vat, and every time it emerges, a UV or laser light source hardens the resin on the platform into layers that form a component, according to the design parameters of the system's connected CAD software. When assembly is complete, the system removes the product from the vat, which has been drained of excess resin.
Objects additively manufactured through vat polymerization may need more post-processing than parts made through other AM methods: Further UV or laser exposure can be necessary to harden the component effectively. Also, during the build, support structures sometimes must be in place on the platform so the hardening resin forms the designer's intended shape; if used, these must later be carefully removed from the part. Overall, vat polymerization is a fast and accurate process, but because finished components are not necessarily degradation- or corrosion-resistant, it may be better for prototyping than producing market-ready parts.
Material and binder jetting
Material jetting is perhaps closer in nature to "printing" than most other additive manufacturing methods, even though 3D printing is sometimes used as an overall synonym for AM. Nozzles above a build platform emit liquefied material (usually polymer or wax; occasionally metal) as repetitive drops onto a build platform. UV light hardens the drops as they form layers in line with design parameters and take the planned object's shape. Because material jetting is only compatible with a select few materials, its utility is limited; the method's chief advantage is low waste, which is also helpful for rapid prototyping.
Binder jetting combines powder and liquid, depositing them in alternating layers, and the liquid — typically a polycarbonate or polyamide — functions as an adhesive. The main advantage of this method is that powdered metal alloys and polymers or ceramics can be used together to create a part, but this is somewhat offset by the lengthy post-processing time required for the liquid layers to cool and form suitable bonds.
Material extrusion
This generally refers to fused deposition modeling (FDM), one of the first additive manufacturing methods to see industrial success. It's also the method best known to consumer users of desk-sized 3D printers. Polymer or plastic is drawn into the printer, heated and finally deposited in layers through a nozzle, which automatically adjusts throughout the process to meet design specifications.
Material extrusion via FDM can only print with polymer or plastic. Although an FDM industrial printer is compatible with ABS plastic, which can be the basis for sturdy parts, it's slow and only moderately accurate when compared to other additive manufacturing processes. This is the main reason why companies producing FDM printers pivoted sharply, if not entirely, to the consumer market.
Sheet lamination
An additive manufacturing method exclusive to sheet metal and paper, sheet lamination has limited applications, but can be useful for high-speed, low-cost prototype fabrication.
The metal version of this process is called ultrasonic additive manufacturing (UAM), and involves the ultrasonic welding of metal sheets (usually aluminum, copper, stainless steel or titanium). By contrast, laminated object manufacturing (LOM) involves layering paper sheets with adhesive. Both are only suitable for creating part models. That said, UAM's ability to use multiple metals may help designers and engineers simulate how additively manufactured metal parts made of copper, for example, might be compatible alongside other metals in specific industrial applications.
Directed energy deposition
As its name implies, directed energy deposition (DED) typically uses a nozzle mounted atop a highly flexible automated arm to deposit material in layers atop a build platform at the exact same time a heat source is focused on the substance. DED has a number of variations, and can thus use polymer, ceramic or metal material, but its most common subcategory, electron beam melting (EBM) is exclusive to metal.
While impractical for part production, this method can be useful as an additive technique for component repair, due to the flexibility of its nozzle. However, the high-speed repair DED is capable of will reduce accuracy, and if accuracy is a priority, engineers must sacrifice speed.
Powder bed fusion
Some of the most common additive manufacturing techniques fall under the umbrella of powder bed fusion, including SLS, selective heat sintering (SHS), selective laser melting (SLS) and EBM. These methods' shared element is a reliance on powdered polymers or metals: Powders are taken from a container below or next to the build platform (bed) with a roller or blade in layers and then heated to solidify.
Key differences between the powder bed fusion methods are as follows:
-
SLS fuses (sinters) polymers or plastics into finished parts.
-
Direct metal laser solidification (DMLS) is the SLS variation used for powdered metals.
-
SHS uses a thermal print head to sinter the layers in the powder bed together.
-
EBM only works with metal powders under vacuum conditions, which allow the electron beam to fuse the layers in the powder bed.
Powder bed fusion is somewhat slower than other additive manufacturing methods and requires significant power usage. But because it's mostly reserved for industrial applications, these issues aren't necessarily problematic. The laser-based variants of this technique are also arguably more capable of reliably producing functional, market-ready parts as opposed to models or prototypes.
EOS’ contributions to AM technologies
Industrial 3D printing systems available from EOS use the SLS and DMLS variations of powder bed fusion for polymer and metal powders, respectively. In fact, EOS not only helped popularize SLS for industrial applications, but specifically invented DMLS for metal 3D printing almost 30 years ago. Back when few thought additive manufacturing with metals was even plausible, our engineers and experts were refining our tools and methods to make it a reality.
Innovation across the board
EOS' reputation in the additive industry doesn't rest solely on the powder bed fusion methods we refined, the 3D printing systems we developed or the polymer and metal additive manufacturing materials we created in-house. We take a holistic approach to additive manufacturing and offer trusted services to support it alongside our hardware and software, including project consulting from the award-winning Additive Minds team and 3D printing courses to help additive novices become designers and refine engineers' skills into expertise.
Contact an Additive Minds expert to learn more, no matter where you are on your journey into the world of additive manufacturing.